By Dr. Thomas T. Yamashita
Introduction – The Frustration of Duplicating Yields from Virgin Soils
In the course of my research, I have long taken note of the fact that in the great majority of cases, the yields and quality achieved on virgin ground is moderately to significantly higher especially in the first two years of cropping over that occurring on continuously cropped soils. Many may find this hard to believe when reviewing mineral analyses from cropped soils, which by standards of soil chemistry tend to be consistently superior to virgin soils.
Many years ago, I conducted chemical analyses of various samples of continuously cropped and virgin soils. The cropped soils were consistently superior in mineral constitution. However, the virgin soils were consistently higher in overall microbial population levels, and in the diversity of microbial species. In addition, one could detect that virgin soil featured a mellow texture, versus the more compact and difficult to work texture typical of cropped soil.
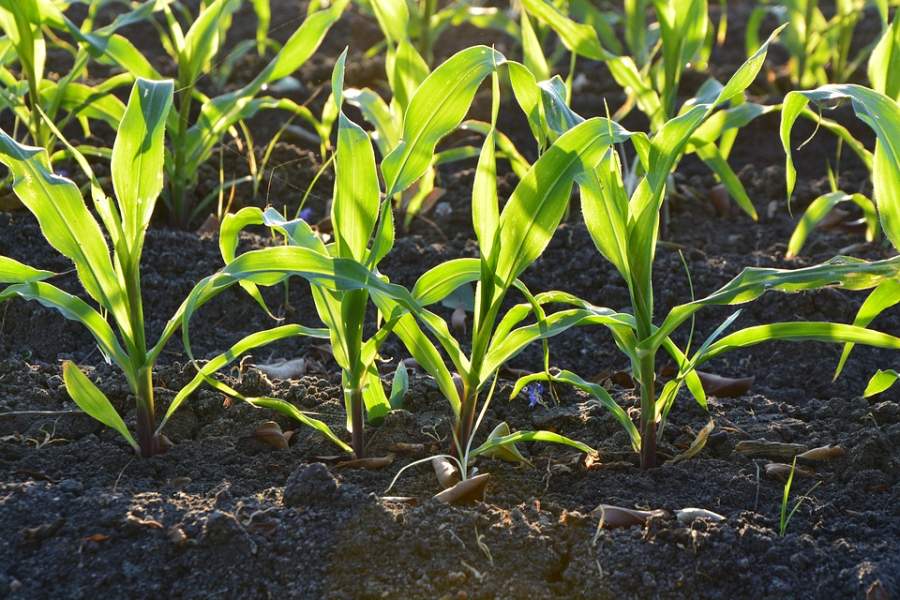
Indeed, in years past farmers recognized the merits of amending their soils with animal manures, as well as green manures. Further, growers in tune with soil conditioning practiced crop rotations—wheat and corn would be rotated with potatoes, and beans and barley would be rotated with broccoli or cauliflower. Those with permanent crops, such as orchards or vineyards would spread manure and allow groundcover to flourish before incorporating this biomass back into the soil.
But as the trends of agricultural fertilization shifted from organic to defined blends, more and more farmers focused entirely upon nitrogen, phosphorus, potassium, and addition of other defined elements. In addition, as profit margins were squeezed, farmers were forced to move away from rotations and other soil-building programs, and instead plant back-to-back high margin crops, attempting to mitigate the build-up of harmful pathogens with fumigation- and fertilization-based solutions, where disease-free, sterile, and fertilizer-rich soil systems became the norm. But in the last half century, regulatory restrictions on the use of pesticides and fumigants have reduced the number of such tools available to growers. Once ubiquitous chemical solutions, such as DBCP, EDB, D-D, and PDB have been banned from further use.
The loss of these chemical crutches has in recent years resulted in a resurgence of interest in conventional soil-building programs utilizing animal manures, green manures, rotations, the use of various types of compost solids, compost tea, preferred microbial strain inoculations, and the introduction of complex liquid carbon sources for proliferating indigenous species of microbes. Numerous microbial companies and products have emerged in recent decades, each touting broad spectrum and miraculous benefits.
There is no question that the Age of Soil Microbiology is upon us. However, the agricultural public is literally being bombarded with microbial technologies and products. Thus farmers, PCAs, chemical distributors, and other agriculturists are being asked to evaluate and make decisions based upon a relatively nebulous and nascent science. Indeed, while the great majority of us have been exposed to soil science and soil chemistry, it is rare to find those who have been formally trained nor introduced at all to the science of soil microbiology.
In this publication, we will introduce soil microbiology and key principles thereof which will form the foundation of this important and budding science as an integral and essential part of sound agricultural practices.
The Players in the Soil
Although oftentimes “out of sight, out of mind,” the soil environment is teeming with activity and numerous organisms. Most organisms range in size from visible (“macroscopic”) to invisible (“microscopic”), and in complexity from single-celled to complex multicellular organisms. Table 1 gives a hint of the magnitude of diversity that can be found in a piece of soil, and the staggering magnitude of these populations.
Table 1 – Organisms Found in a 3’ x 3’ x 6” Volume of European Grassland
Organism | Scientific Group | Numbers Found |
Macroscopic | ||
Earthworms | Lumbricidae | 30 – 2,000 |
Potworms | Enchytraeidae | 200 – 20,000 |
Slugs and Snails | Mollusca | 100 – 8,500 |
Millipedes and Centipedes | Diplopoda and Chilopoda | 900 – 1,700 |
Woodlice | Isopoda | 100 – 400 |
Spiders | Araneida | 180 – 840 |
Beetles and Larvae | Coleoptera | 500 – 1,000 |
Fly Maggots | Diptera | 200 – 1,000 |
Ants | Hymenoptera | 200 – 500 |
Springtails | Collembola | 10,000 – 40,000 |
Mites | Acarina | 20,000 – 120,000 |
Moss | Thallophyta: Bryophyta | 400 – 10,000 |
Microscopic | ||
Bacteria | Protista: Schizomycetes | 940 billion – 9.8 trillion |
Actinomycetes | Protista: Actinomycetes | 1.8 billion – 5.264 trillion |
Fungi | Mycota | 10 billion – 1 trillion |
Protozoa | Protista: Protozoa | 583 million – 4.59 billion |
Algae | Thallophyta | 3.76 billion – 11 billion |
Nematodes | Nemata | 1.8 million – 9.4 billion |
Tardigrades | Tardigrada | 1.8 million – 53 million |
Thus, this representative 4.5 cubic feet of grassland soil hosts an amazing array and number of diverse macroscopic and microscopic organisms. Despite their inconspicuous nature, the microorganism community is diverse and numerous. It is said about nematodes, for example, that if all the soil were removed from the earth, the mass of nematodes would still provide a noticeable outline of the Earth’s sphere.
To give you an idea of the scale of this on a more comprehensible level: During field experiments in which I activated the soil bacterial populations to unusually high levels, it was calculated that within 3 weeks a bacterial biomass developed which was estimated at 6 tons per acre furrow slice (1 acre, 6” deep). An additional 2.2 tons of fungal biomass had also developed.
The Overall Picture of the Microorganism Community
Now that we have had a glimpse of the possible diversity and population of microorganisms, we need to take the next step in defining some spatial and chronological order to the community. We should first begin by understanding the broad and general relationships between organism groups. Let’s start by drawing some understanding of animal and plant life on the surface of the Earth.
Table 2 and 3 shows the classification of all plant and animal life on earth based on their habit and ecological role. This includes – basic foundational organisms such as green plants, secondary grazers such as cattle that graze on foundational organisms, predators such as mountain lions that lack the ability to digest and assimilate basic foundational organisms (i.e. plants) and thus must rely upon preformed animal tissues created by the secondary grazers, and detritus scavengers such as dung beetles and flies that utilize waste products from secondary grazers and predators.
Table 2 – Earth-Dwelling Organisms Types, Habit, and Ecological Role
Organism Type | Examples | Basic Habit | Product Tissues |
Basic Foundational Organism | Plants (Alfalfa, Corn) | Processes CO2 + H20 + minerals to → | Plant Tissues |
Secondary Grazers | Herbivores (Cattle, Sheep) | Graze on plant tissues, convert to → | Animal Tissues – 1, Animal Waste – 1 |
Predators | Carnivores (Lions, Wolves) | Prey on herbivores and convert to → | Animal Tissues – 2, Animal Waste – 2 |
Detritus Scavengers | Waste Feeders (Dung Beetles, Flies) | Feed on animal wastes, converting to → | Animal Tissues – 3, Animal Waste – 3 |
Secondary Predators | Secondary Carnivores (Shrews, Birds) | Prey on detritus scavengers, convert to → | Animal Tissues – 4, Animal Waste – 4 |
The ecological continuity of each organism type is one of interrelationship and interdependence, with multiple recycling processes capturing tissues and waste materials and reusing them to produce new complex tissues. A dynamic continuum develops, whereby carbon and energy are reconverted or recycled in a complex but repetitive process.
But for all this to work, basic foundational organisms must thrive, as they constitute the first essential step which initiates this series of ecological relationships. With the advent of man and agriculture, we have now added another step in this process, in which we provide assistance to enhance the growth of basic foundational organisms, increasing the momentum of this continuum and the diversity and population of the organisms which comprise it.
Now, let’s return to soil microbiology and draw some analogies between the various microorganisms listed in Table 1 and the categories of organisms defined in Table 2:
Table 3 – Grouping of Microorganisms According to Ecological Roles
Basic Found. Org. | Secondary Grazers | Predators | Detritus Scavengers | Secondary Predators |
Bacteria | Protozoa | Tardigrades | Bacteria | Tardigrades |
Actinomycetes | Plant-Parasitic Nematodes | Mites | Actinomycetes | Mites |
Fungi | Free-Living Nematodes | Spiders | Fungi | Spiders |
Algae | Slugs and Snails | Millipedes | Springtails | Millipedes |
Moss | Ants | Centipedes | Mites | Centipedes |
Earthworms | Beetles | Fly Maggots | Beetles | |
Potworms | Bacteria | Earthworms | Bacteria | |
Woodlice | Actinomycetes | Potworms | Actinomycetes | |
Beetles | Fungi | Woodlice | Fungi | |
Bacteria | Protozoa | Beetles | Protozoa | |
Actinomycetes | Free-Living Nematodes | Free-Living Nematodes | Free-Living Nematodes | |
Fungi | Ants | Protozoa | Ants |
Notice how there is repetitive overlap in microorganism types within various ecological groupings—as can be seen with actinomycetes, nematodes, and fungi—due to their ability to harvest various nutritional elements from the atmosphere, waste products, and complex organisms. For example, some types of free-living nematodes can feed on fungi or bacteria, act as predators of earthworms and other nematodes, and be parasites of insects.
Initiating the Microbial Activity of the Soil
What is important to reemphasize is that basic foundational organisms are the first wave of microbial biomass in the ongoing ecology of the soil. Without this group’s proliferation, the succeeding waves of microorganism classes move into the scene slowly, or not at all. A key question to ask then is, “What contributes to the proliferation of basic foundational organisms?” We must realize that the answers to this question constitute the foundations of microbial soil enhancement. Because of the diversity of these organisms with varying types of basic food and mineral requirements, we can generalize some key components, as seen in Table 4.
Table 4 – Components Necessary to Proliferate Basic Foundational Organisms
Component(s) | General Sources |
Water | Rains, floods, irrigation, subterranean water |
Essential Inorganic Elements (N, P, K, Ca, Mg, Na, etc.) | Atmospheric nitrogen, natural deposits, fertilizers, water |
Organic carbon compounds (cellulose, carbohydrates, proteins, etc.) |
In our farming operations we generally supplement rainfall with periodic irrigation. Thus, most of us consider the requirement of water as a given. Further, most agronomic soils are supplied, either inherently or through fertilizer additions, with inorganic minerals. However, the factor that is minimal to lacking in most cases is the addition of organic carbon compounds. Where crop rotations and incorporation of cover crops are practices, soils tend to receive greater quantities of organic carbon. However, permanent crops such as vineyards and orchards characteristically institute herbicide stripping and “clean culture.” That is, there is minimal replenishment of the soil with organic carbon.
If foundational organisms are to be proliferated, and thus kickstart the Soil Microbial Ecological Cycle (SMEC) that produces healthy, supple soils, the addition of organic carbon compounds becomes imperative. Table 5 provides a comparison between the basic constitution of a typical microbial cell versus that of a plant. An examination of this table should serve to emphasize why the presence of organic carbon compounds are critical to producing healthy soils.
Table 5 – The Approximate Elemental Constitution of an Average Microbial Cell and Average Plant
Microbial Cell | Plant | ||
Element | Percentage of Dry Weight | Element | Percentage of Dry Weight |
Carbon | 50% | Carbon | 43% |
Oxygen | 20% | Oxygen | 43% |
Nitrogen | 14% | Nitrogen | 2% |
Hydrogen | 8% | Hydrogen | 6% |
Phosphorus | 3% | Phosphorus | 0.2% |
Sulfur | 1% | Sulfur | 0.2% |
Potassium | 1% | Potassium | 2% |
Sodium | 1% | Sodium | 0.01% |
Calcium | 0.5% | Calcium | 2% |
Magnesium | 0.5% | Magnesium | 0.4% |
Chlorine | 0.5% | Chlorine | 0.1% |
Iron | 0.2% | Iron | 0.02% |
Others | 0.3% | Others | 1% |
It should be evident that there are a number of similarities between the constitutions of microbes and plants. Thus, the addition of plant materials and their byproducts or extracts can provide a balanced array of compounds and minerals necessary to help initiate the SMEC. In recent years the agricultural marketplace has been bombarded with various companies which tout microbial inoculants, compost teas, and enzyme solutions to initiate SMEC. While these materials may have their place in agriculture, the primary catalyst which must be present to initiate SMEC is available carbon compounds and minerals.
One important difference between microbial biomass and plant biomass is the carbon-nitrogen ratio (C:N). In microbes, this C:N ratio is close to 4:1, whereas in plants this ratio is usually greater than 20:1. When dealing with dried wheat or barley straw, the ratio climbs to greater than 90:1. Most hardwoods have a C:N ratio exceeding 200:1. Legumes generally host a C:N ratio between 14:1 and 20:1. The point is that in order to encourage efficient transformation of organic carbon sources to microbial biomass, one must balance the C:N ratios of their organic carbon addition in near approximate to C:N ratios held by the microbes. Thus, farmers cognizant of this need will oftentimes spread a nitrogen-based fertilizer before incorporation stubble or green manure. Those who do not add additional nitrogen will observe a dramatic reduction in both the rate of tissue decomposition as well as absolute decomposition itself. How often, for example, have we observed a peach orchard in which shredded prunings have been incorporated in mid-spring, only to find the emerging foliage turn yellow from lack of nitrogen, literally robbed from the trees by the activated microbes? Therefore, As a general rule, then, getting the C:N ratio close to 20:1 allows for effective decomposition and conversion of plant to microbial biomass. A more precise formula can be seen in Table 6.
Table 6 – Steps in Priming Organic Carbon Sources to Facilitate Efficient Conversion to Microbial Biomass
Step | Method |
1. Estimate the carbon biomass. | Weigh the plant biomass from a known sample area and adjust to produce estimate for 1 acre. |
2. Assign ~40% carbon loss to CO2. | Subtract 40% of the estimated biomass weight from the total. |
3. Divide the remaining biomass by a factor of 20 (from 20:1). | Divide the remaining 60% of the estimated carbon biomass by 20. |
4. The quotient gives the minimum units of N to add. | Apply the calculated N to the field before incorporating the utilized carbon source. |
An example of how to apply the method described in Table 6 is as follows:
- Estimating plant tissue biomass: Biomass from 1/100th of an acre is found to weigh 20 pounds. Multiplying by 100 produces an estimate of 2,000 pounds of biomass per acre.
- Accounting for carbon loss: 40% of 2,000 pounds is 800 pounds. 800 is subtracted from 2,000, producing an estimate of 1,200 pounds.
- Accounting for 20:1 C-N ratio: The remaining biomass of 1,200 pounds is divided by 20, providing a final figure of 60.
- Application: Thus, it is necessary to apply a minimum of 60 units of nitrogen, equivalent to 28.2. gallons of CAN-17, or 17 gallons of UAN-32.
Recapping the General Nature of Soil Microbial Biology
The diversity and mass of the developed microbial community will vary directly with the quantity and quality of the consumable materials provided. Cyclic regeneration and transformations of the original carbon-mineral food base creates a dynamic, gradually dwindling microbial biomass. During metabolic activity of the microbial populations, that energy is being extracted from carbon compounds and converted into various forms of microbial tissue and products. Metabolic conversions of carbon compounds frequently result in the generation of carbon dioxide (recall the 40% loss of carbon via CO2 during the decomposition process). Thus, it’s important to periodically replenish the system with maintenance applications of what might be called “primary microbial foods.” Lack of attention to this factor is one of the main reasons for the high incidence of sterile soils on many American farms. The condition is aggravated with commonly instituted practices of fumigation, herbicide stripping, orchard removal of prunings, and many more.
Aside from our overall findings that microbially enriched soils produce higher yields and quality crops, there are numerous additional benefits which may further explain these improvements. Some of these benefits include:
Enhanced release of minerals otherwise locked in the soil, due to:
- The microbial production of organic acids, which act to:
- Create localized pockets of buffered pH drop
- Chelation or ‘complexing’ of minerals
- Direct solubilization of tied-up minerals through microbial extracts and exudates
- Improved water-holding capacity of soils, assisting in hydration and solubilization of minerals.
Increased root volume, due to:
- Improvements in the timely release of otherwise tied-up minerals
- Antagonism of soil-borne pathogens
- Improved water infiltration rates and aeration factors
Increased rates of water infiltration, due to:
- Copious production of gums, which bind separate clay particles into aggregates
- Greatly enhanced and evenly distributed microbial biomass within the soil (“bulking”)
Reductions in disease inoculum, due to:
- Substrate or enzymatically advantaged competition for variable food sources
- By-product inhibition (e.g. antibiotic production by strains of Bacillus or Penicillium)
- Mycoparasitism or the colonization of pathogens directly by antagonists (e.g. Trichoderma spp.)
- Siderophore production or education of chelating agents that aid in competition for key minerals
- Competitive displacement of the pathogen by virtue of combined factors, including rapid growth rates
Summary: The Missing Link
Virgin soils are characteristically lower in soil mineral content, but are known to consistently surpass continuously cropped soils with highly intensified soil fertility programs. Comparative examinations of cropped versus virgin soils indicate that the latter is endowed with superior diversity and number of microbiota. Most of the factors being microscopic, as well as housed in the soil environment, contributes greatly to the inconspicuous and largely unrecognized nature of the benefits of soil microbiota.
Further, most agriculturists are schooled in soil chemistry, but few have been exposed to the subject of soil microbiology. Modern agriculture is still relatively young. Thus, the advent of technologies such as soil fumigation and general soil sterilization methods manifested dramatic growth and yield responses by virtue of the removal of a formidable pathogen or pest. In addition, the introduction of these technologies coincided with increased use of formulated, defined fertilizers. Not too long after, herbicide technology was introduced, and growers with permanent crops developed herbicide stripping, which created an 8- to 10-foot-wide zone of weed-free ground.
The marketplace boomed, and as the pace of life intensified, so did the need for higher production. Fertilizer rates climbed, and many ammonical and urea-based applications acted as sterilants in themselves. Many growers were forced to forego soil-building rotations and the use of cover crops, planting back-to-back, high-margin crops. The complacency we have developed with this arsenal of “magic bullet” chemicals has been challenged many times over. Despite the use of such treatments, which control pests, pathogens, and weeds, combined with intensified soil fertility programs, many growers are experiencing a plateau or outright decline in yield and quality.
Of the many field calls I have had the opportunity to address over the years, a conservative estimate would place 95% of the maladies as originating from one or more of the following:
- Suboptimal nutrition
- Predisposition as a result of a weakened host
- Sterile soil with low natural suppressiveness and heightened pathogen activity
Soil microbiology is a huge missing link in today’s agriculture. If used properly and with pre-characterized specificity for each individual field, intelligently applied microbial technologies have been shown to provide a quantum leap in plant growth and productivity. As agriculturists committed to excellence and a continued search for improvement, we will need to arm ourselves, among others, with the principles of soil microbiology and its related disciplines.